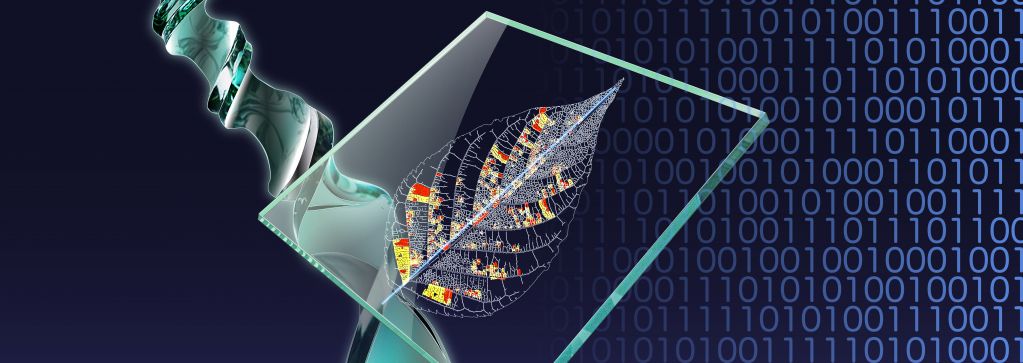
Keynote Speakers
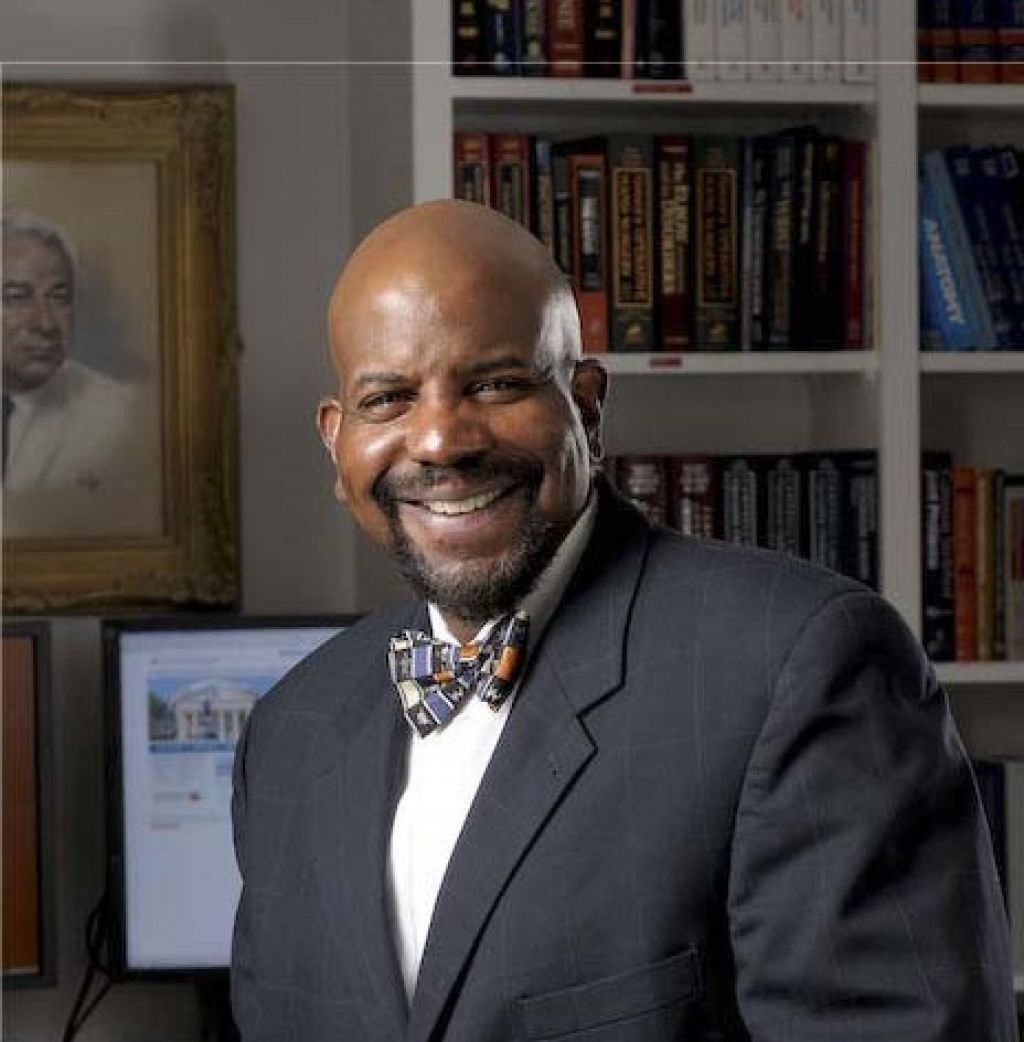
Cato T. Laurencin, MD, PhD
Distinguished Professor of Orthopedic Surgery
Professor of Chemical and Biomolecular Engineering, Materials Science and Engineering, Biomedical Engineering
Director, The Raymond and Beverly Sackler Center for Biomedical, Biological, Physical and Engineering Sciences
Chief Executive Officer, The Connecticut Convergence Institute for Translation in Regenerative Engineering, The University of Connecticut
Cato T. Laurencin, MD, PhD is the Founder of the field of Regenerative Engineering. He is the Editor-in-Chief of the Journal Regenerative Engineering and Translational Medicine and Founder and President of the Regenerative Engineering Society, now a community of the American Institute of Chemical Engineers (AICHE). The AICHE Foundation created and endowed the Cato T. Laurencin Regenerative Engineering Founder’s Award in his honor. Dr. Laurencin’s work ranges from basic science, to applied science, to engineering, to biomedical translation. His awards range from the Priestley Medal (highest honor) of the American Chemical Society, to the Von Hippel Award (highest honor) of the Materials Research Society, to the Founder’s Award of the Society for Biomaterials, to the Founder’s Award of the American Institute of Chemical Engineers, to the Pritzker Award (highest honor) of the Biomedical Engineering Society, to the Pierre Galletti Award (highest honor) of the American Institute for Medical and Biological Engineering, to the James Bailey Award (highest honor) of the Society for Biological Engineering. He has also earned principal honors in orthopaedic surgery (The Nicolas Andry Award from the Association of Bone and Joint Surgeons, the Kappa Delta Award from the American Academy of Orthopaedic Surgeons, the Marshall Urist Award from the Orthopaedic Research Society and the Distinguished Contributions to Orthopaedics Award from the American Orthopaedic Association).
In science, engineering, medicine, and innovation, he is an elected member of the National Academy of Sciences, an elected member of the National Academy of Engineering, an elected member of the National Academy of Medicine, and an elected Fellow of the National Academy of Inventors. He is the first surgeon in history to be elected to all four national academies. Dr. Laurencin received the Philip Hague Abelson Prize, the highest honor of the American Association for the Advancement of Science, for "signal contributions to the advancement of science in the United States" for his work in Regenerative Engineering. He is the first person to receive both the oldest/highest award from the National Academy of Engineering (the Simon Ramo Founder's Award) and one of the oldest/highest awards of the National Academy of Medicine (the Walsh McDermott Medal). In innovation, Dr. Laurencin was awarded the National Medal of Technology and Innovation, America's highest honor for technological advancement, by President Barack Obama in ceremonies at the White House.
Dr. Laurencin received his B.S.E. in Chemical Engineering from Princeton University, his M.D., Magna Cum Laude from the Harvard Medical School, and his Ph.D. in Biochemical Engineering/Biotechnology from the Massachusetts Institute of Technology. He serves as the University Professor at the University of Connecticut (one of two currently at the school).
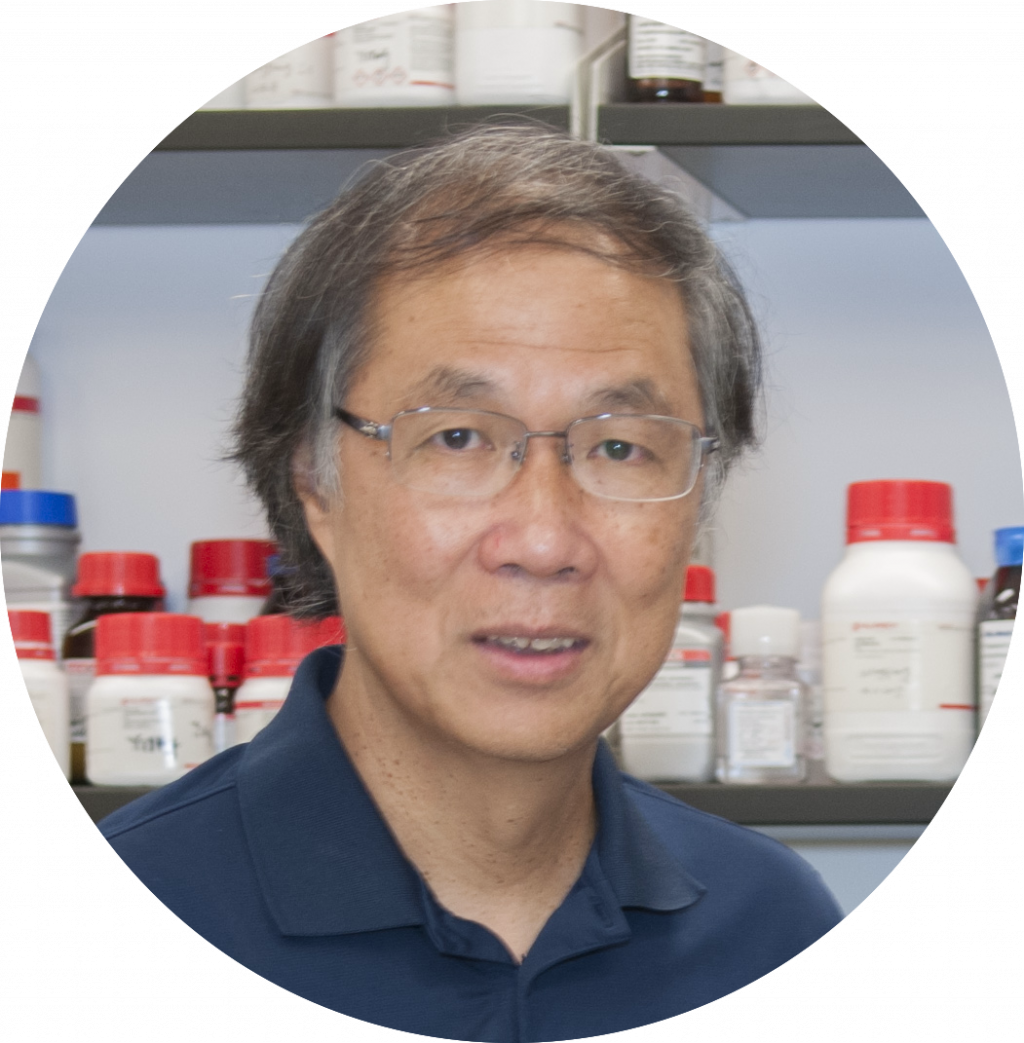
Kam W Leong, PhD
Samuel Y Sheng Professor, Department of Biomedical Engineering, Columbia University, New York.
Kam W. Leong is the Samuel Y. Sheng Professor of Biomedical Engineering at Columbia University, where he focuses on three major research directions: 1) Nonviral gene editing in vivo; 2) Biomaterials-assisted modulation of inflammation; 3) Human-tissue chips for disease modeling and drug screening. He has published ~500 manuscripts and holds more than 60 issued patents. He is the recipient of the Founder’s Award of the Society for Biomaterials and the IEEE-EMBS Technical Achievement Award in 2022, Editor-in-Chief of Biomaterials, and a member of the USA National Academy of Inventors, the USA National Academy of Engineering, and the USA National Academy of Medicine.
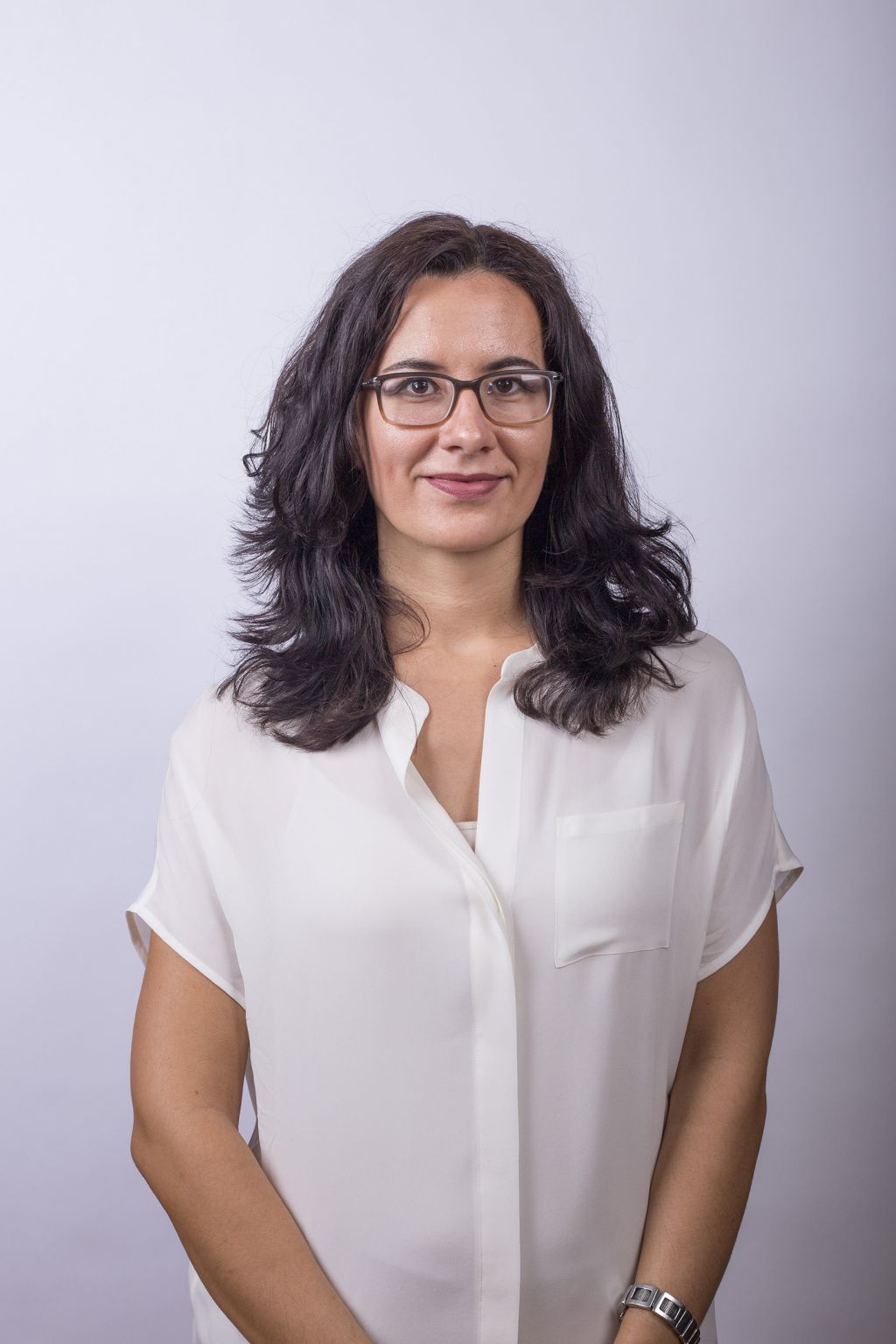
Milica Radisic, PhD
Professor, University of Toronto, Canada
Research Chair in Organ-on-a-Chip Engineering, Senior Scientist, Toronto General Research Institute
Dr. Milica Radisic is a Professor at the University of Toronto, Canada Research Chair in Functional Cardiovascular Tissue Engineering and a Senior Scientist at the Toronto General Research Institute. She is also Director of the NSERC CREATE Training Program in Organ-on-a-Chip Engineering & Entrepreneurship and Director of Ontario-Quebec Center for Organ-on-a-Cho Engineering. She obtained B.Eng. from McMaster University, and Ph.D. form the Massachusetts Institute of Technology. She is a Fellow of the Royal Society of Canada-Academy of Science, Canadian Academy of Engineering, the American Institute for Medical & Biological Engineering and Tissue Engineering & Regenerative Medicine Society. She received numerous awards and fellowships, including MIT Technology Review Top 35 Innovators under 35. She was a recipient of the Queen Elizabeth II Diamond Jubilee Medal in 2013, NSERC E.W.R Steacie Fellowship in 2014, YWCA Woman of Distinction Award in 2018, OPEA Research & Development Medal in 2019 and Killam Fellowship in 2020 to name a few. Her research focuses on organ-on-a-chip engineering and development of new biomaterials that promote healing and attenuate scarring. She developed new methods to mature iPSC derived cardiac tissues using electrical stimulation. Currently, she holds research funding from CIHR, NSERC, CFI, ORF, NIH, and the Heart and Stroke Foundation. She is an Associate Editor for ACS Biomaterials Science & Engineering, a member of the Editorial Board of Tissue Engineering, Advanced Drug Delivery Reviews, Regenerative Biomaterials, Advanced Biosystems, Journal of Molecular and Cellular Cardiology and eLife. She serves on review panels for Canadian Institutes of Health Research and the National Institutes of Health. She is actively involved with BMES (Cardiovascular Track Chair and TERMIS-AM (Council member, Chair of the Membership Committee). She was a co-organizer of a 2017 Keystone Symposium, “Engineered Cells and Tissues as Platforms for Discovery and Therapy”. She served on the Board of Directors for Ontario Society of Professional Engineers, Canadian Biomaterials Society and McMaster Alumni Association. Her research findings were presented in over 200 research papers, reviews and book chapters with h-index of 67 and over 17,400 citations. Her publications appeared in Cell, Nature Materials, Nature Methods, Nature Protocols, Nature Communications, PNAS etc. In 2014, she co-founded an award winning company TARA Biosystems that uses matured human engineered heart tissues in drug development. TARA was acquired in April 2022, by Valo an AI company. In 2017, she founded Quthero Inc, a company focused on disrupting the skin regeneration and a medical esthetics market through the use of proprietary Q-gel to promote scar-free wound healing.
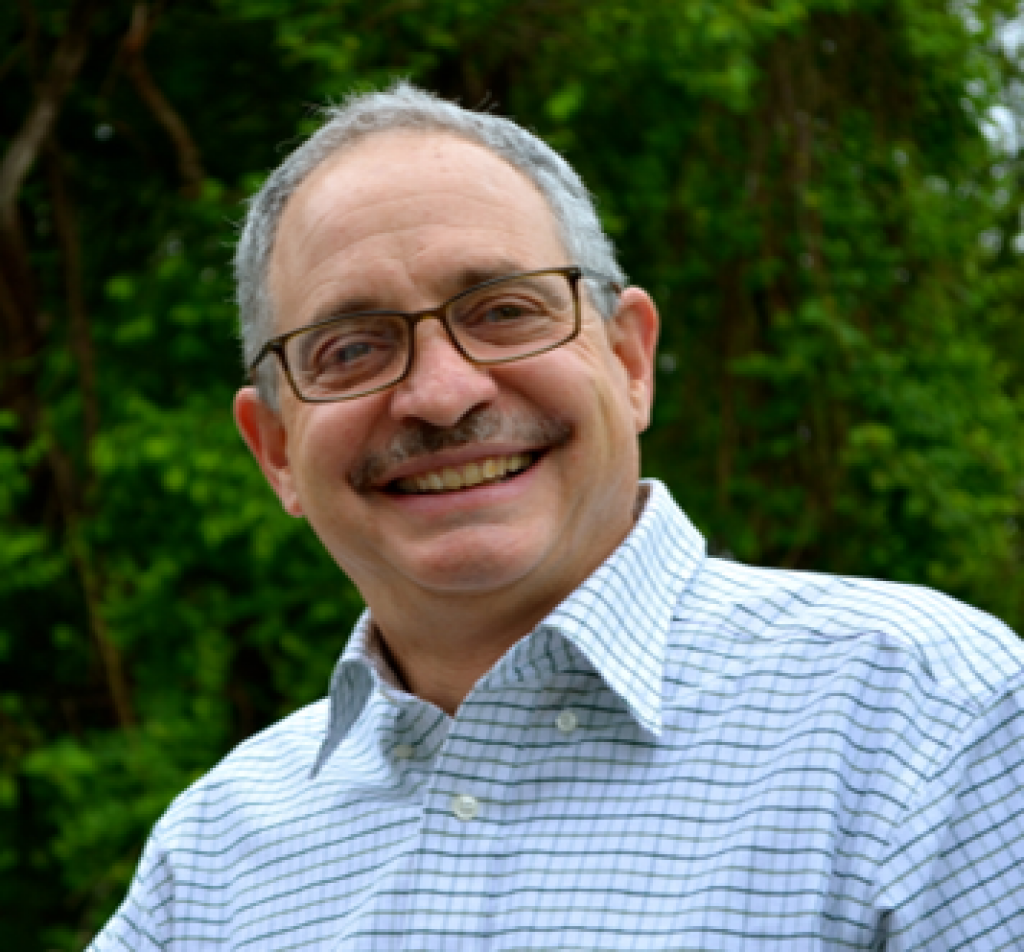
David L. Kaplan, PhD
Stern Family Endowed Professor of Engineering, Professor & Chair, Department of Biomedical Engineering, Distinguished University Professor, Tufts University
David Kaplan is the Stern Family Endowed Professor of Engineering at Tufts University, a Distinguished University Professor, and Professor and Chair of the Department of Biomedical Engineering. He also holds faculty appointments in the departments of Chemical and Biological Engineering, Chemistry, Biology and in the School of Medicine. His research focus is on biopolymer engineering, tissue engineering, regenerative medicine and cellular agriculture. He has published over 1,000 peer reviewed papers, is editor-in-chief of ACS Biomaterials Science and Engineering and he serves on many editorial boards and programs for journals and universities. He has received awards for his research and teaching and is an elected Fellow of the American Institute of Medical and Biological Engineering and the National Academy of Engineering.
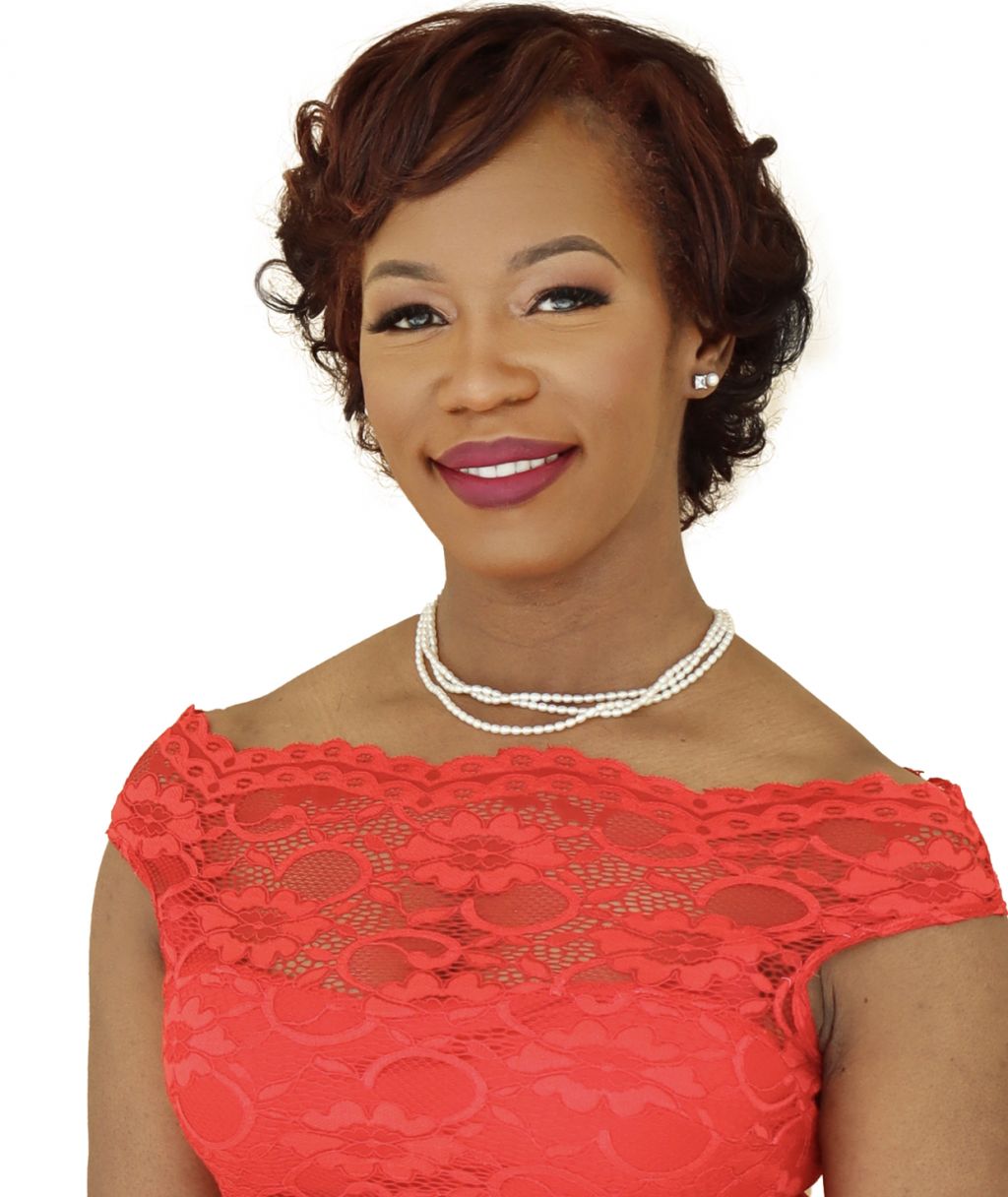
Trivia Frazier, PhD
Co-Founder, President, and CEO, Obatala Sciences
Dr. Trivia Frazier is the Co-Founder, President, and CEO of Obatala Sciences, Inc. She is an experienced stem cell biology and tissue engineering researcher and biotech business professional with over 15 years of experience in biomedical sciences and management. She holds bachelor's degrees in Physics and Biomedical Engineering, an MBA, and a PhD in Biomedical Sciences. Dr. Frazier completed her postdoctoral work under an NIH-funded fellowship at Pennington Biomedical Research Center and the Tulane Center for Stem Research and Regenerative Medicine. She has collaborated with national and international key opinion leaders and held Academic Chair appointments prior to her leadership role at Obatala. Under her leadership, the Obatala team has received federal funding through SBIR Phase I awards from the National Center for Advancing Translational Sciences to commercialize the Obatala suite of human-derived stem cells and 3D cell culture products. These products are used to model human adipose tissue from patients of varying demographics.
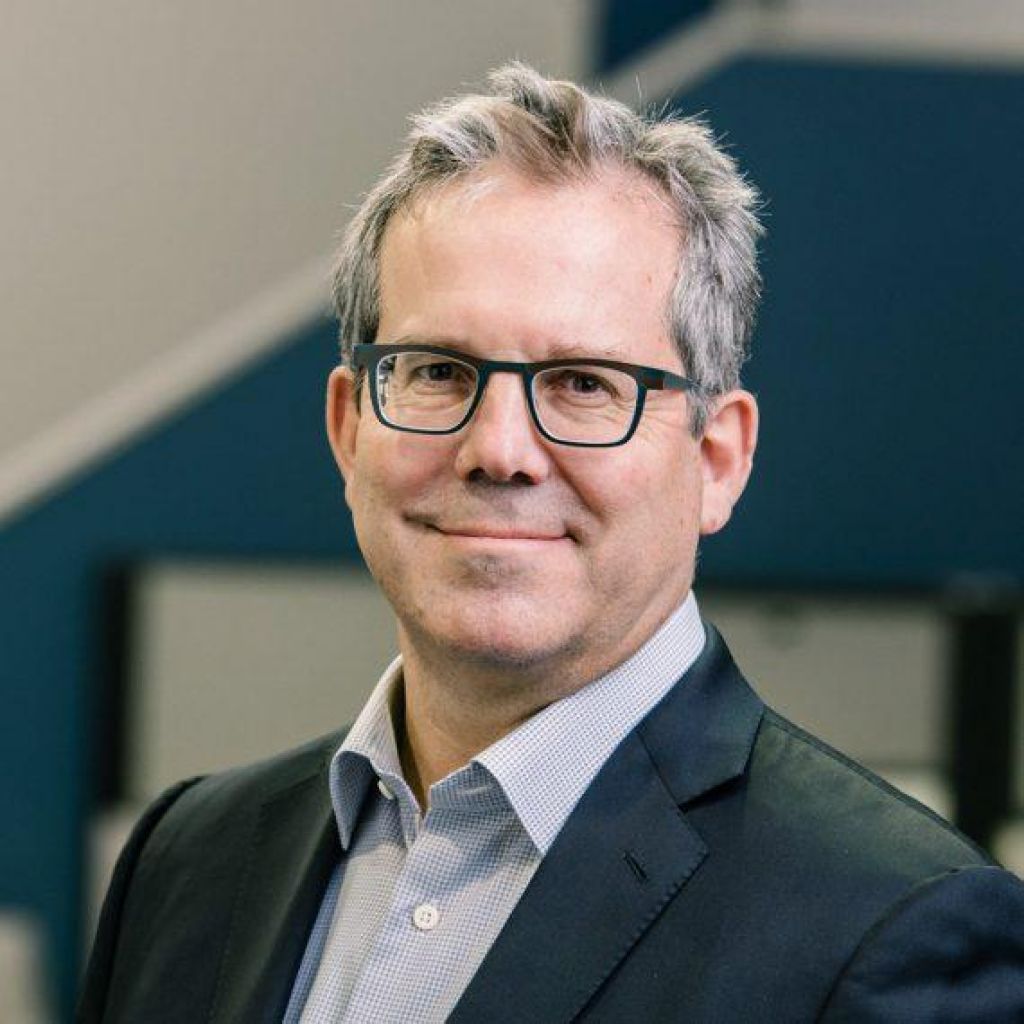
Geoffrey C. Gurtner, MD, FACS
Chair, Department of Surgery, Professor of Surgery and Professor of Biomedical Engineering, University of Arizona College of Medicine – Tucson
Geoffrey Gurtner, MD, FACS is the Professor and Chair, Department of Surgery/Biomedical Engineering at University of Arizona. Prior to his current position, Dr. Gurtner was the Johnson & Johnson Distinguished Professor of Surgery, co-director of the Hagey Laboratory for Pediatric Regenerative Medicine, professor of bioengineering, professor of materials science and engineering, executive director of the Stanford Advanced Wound Care Center and inaugural vice chair for Innovation in the Stanford Department of Surgery. Dr. Gurtner is internationally recognized as a highly accomplished clinician and prolific researcher, author, inventor, and entrepreneur. He has been awarded more than $27 million in federal research funding and holds 35 patents. He also has founded three venture capital-backed life sciences companies. He has authored or co-authored 345 peer-reviewed articles and nearly 50 book chapters, and he has edited the two major textbooks in his field. He is the immediate past board president of the Wound Healing Society, the premier scientific organization focused on wound healing. Dr. Gurtner graduated from Dartmouth College and earned his medical degree at the University of California, San Francisco. He completed his internship and surgical residency at Massachusetts General Hospital, Harvard Medical School, followed by a plastic surgery residency at the Institute of Reconstructive Plastic Surgery at NYU Medical Center and a fellowship in oncologic microsurgery at the University of Texas MD Anderson Cancer Center in Houston.
Flash Talks
Xiaojun Lance Lian, PhD. Associate Professor of Biomedical Engineering & Biology
When gene editing meets modified RNA
CRISPR systems have revolutionized biomedical research because they offer an unprecedented opportunity for gene editing. However, a bottleneck of applying CRISPR systems in human pluripotent stem cells (hPSCs) is how to deliver them into cells easily and efficiently. Here, we developed modified mRNA (modRNA)-based CRIPSR systems that utilized Cas9 and p53DD or a base editor (ABE8e) modRNA for the purposes of knocking out genes in hPSCs. ABE8e modRNA was employed to disrupt the splice donor site, resulting in defective splicing of the target transcript and ultimately leading to gene knockout. Using our modRNA CRISPR systems, we achieved 73.3% ± 11.2% and 69.6 ± 3.8% knockout efficiency with Cas9 plus p53DD modRNA and ABE8e modRNA, respectively, which was significantly higher than plasmid-based systems. In summary, we demonstrate that our non-integrating modRNA-based CRISPR methods hold great promise as more efficient and accessible techniques for gene editing of hPSCs.
Amir Sheikhi, PhD. Assistant Professor of Chemical and Biomedical Engineering
Granular Hydrogel Bioinks with Preserved Interconnected Microporosity for Extrusion Bioprinting
Three-dimensional (3D) bioprinting of granular hydrogels comprising discrete hydrogel microparticles (microgels) may overcome the intrinsic structural limitations of bulk (nanoporous) hydrogel bioinks, enabling the fabrication of modular thick tissue constructs. The additive manufacturing of granular scaffolds has predominantly relied on highly jammed microgels to render the particulate suspensions shear yielding and extrudable. This inevitably compromises void spaces between microgels (microporosity), defeating rapid cell penetration, facile metabolite and oxygen transfer, and cell viability. We will discuss how this persistent bottleneck is overcome by programming microgels with reversible interfacial nanoparticle self-assembly, enabling the fabrication of nanoengineered granular bioinks (NGB) with well-preserved microporosity, enhanced printability, and shape fidelity. The microporous architecture of bioprinted NGB constructs permits immediate post-printing 3D cell seeding, which may expand the library of bioinks via circumventing the necessity of bioorthogonality for cell-laden scaffold formation. We envision that NGB will open new opportunities for the 3D bioprinting of microporous scaffolds beyond the traditional biofabrication window to accelerate tissue regeneration.
Scott Selleck, PhD. Professor of Biochemistry & Molecular Biology
A new pathway to suppress neurodegeneration
With our increasingly aging population Alzheimer’s (AD) and other neurodegenerative diseases are a growing health problem and despite 40 years of molecular and genetic studies, there are no pharmaceuticals that arrest or prevent degeneration. This is likely because the focus of pharmacological interventions has been directed at the deposition of protein aggregates, amyloid in the case of AD, that is not necessarily the root cause of cell damage or loss. However, a great deal of study has shown that neurodegeneration is associated with disruptions of other vital processes, including membrane trafficking, mitochondrial function and lipid metabolism. Our work has identified a class of cell surface co-receptors that govern signaling processes which in turn influence all of these fundamental cell biological processes. Heparan sulfate modified proteins are a class of molecules located at the cell surface and in the extracellular matrix that bind secreted protein growth factors and modulate their signaling activity. A number of signaling systems are modulated in this way, including those that direct PI3 kinase/Akt and MAPK pathways. We have discovered that compromising specific steps in heparan sulfate biosynthesis increases autophagy to lysosome trafficking, promotes expansion of the mitochondrial compartment, and reduces the accumulation of intracellular lipid. Altering heparan sulfate chain length or sulfation can suppress cellular deficits in a number of models of human neurodegenerative disease, including Parkinson's and Alzheimer's. Small molecule screening directed at these targets is in progress.
Cunjiang Yu, PhD. Associate Professor of Engineering Science & Mechanics; Biomedical Engineering
Rubbery Electronics: A New Bioelectronics Platform for Biology
The distinction between conventional electronics and soft human body and organs poses a grand challenge in the broad medical field. Our solution to address the challenge through the invention of a new class of electronics, namely rubbery electronics, which is constructed all based on elastic rubber electronic materials with tissue-like softness and mechanical stretchability to allow seamless integration with soft deformable tissues and organs. This presentation will briefly introduce our recent development rubbery electronics, such as rubbery transistors, integrated circuits, sensors and actuators, and their unique capabilities in bio-integrated usages.
Jing Du, PhD. Associate Professor of Mechanical Engineering
Multi-Dimensional and Multi-Scale Mechanical Characterization of Biomaterials, Biological Tissues, and Cells
The biomechanical compatibility of the biomaterials, biological tissues, and cells is one of the important factors for effective tissue regeneration. We have developed several mechanical testing methods to characterize the mechanical properties and mechanical behaviors (deformation, damage, and fracture) of biomaterials, biological tissues, and cells in multiple dimensions and across multiple length scales. (1) Using incremental three-point bending coupled with micro X-ray computed tomography (micro-CT), the 3D internal crack initiation and crack propagation behaviors inside polymer-ceramic composites were revealed. Combining strain mapping using digital volume correlation and fracture mechanics theories, the crack growth was quantified. (2) Using compression coupled with micro-CT, the 3D full-field strain in peri-implant bone was mapped. The high strain concentration appeared in the clinically observed bone resorption region. Micro-CT images were converted to finite element models to compute and predict outcomes for the alternative implant placement plans. (3) Using the PeakForce quantitative nanomechanical mapping mode of atomic force microscope, the elastic modulus maps were obtained for structures in bone across multiple length scales, including submillimeter osteons and interstitial bone, micrometer-scale lamellae and cement lines, and submicron scale mineralized collagen fibril bundle and extrafibrillar matrix. (4) The deformation and strain over time inside single cells and cell clusters were characterized by using digital image correlation of videos taken by optical microscopes.
Urara Hasegawa, PhD. Assistant Professor of Materials Science & Engineering
Polymeric micelles for delivery of gasotransmitter hydrogen sulfide and their potential application in tissue engineering.
Hydrogen sulfide (H2S) is an important signaling molecule that is involved in many physiological processes in human body. With the discovery of its biological significance in cardiovascular, nerve and immunological systems, the potential for its use as therapeutic agent has attracted growing attention. One of the challenges in H2S-based therapy is the lack of the delivery systems that can deliver H2S at the target site in a controlled manner. To address this issue, we developed polymeric micelles bearing H2S donating anethole dithiolethione (ADT) groups and evaluated their H2S release profiles as well as biological activities. In this presentation, we will present design, synthesis and characterization of polymeric micelles with ADT groups. Proangiogenic activity as well as protective effect against ischemic cell damage of the micelles will also be discussed.
Yogendra Pratap Singh, PhD. Postdoctoral scholar
Islets Bioprinting for Fabrication of Pancreatic Devices for Type 1 Diabetes
Type-I diabetes is an autoimmune disease that develops after the auto-destruction of beta-cells in the pancreas. Contemporary treatments include subcutaneous insulin injections and pancreas or islet transplantation. Both transplantation approaches are however limited by the chronic shortage of donor pancreases. In addition, loading human islets into implantable devices in a sufficient density for effective cure has been a long-standing problem. Herein, we aim to 3D bioprint tissue engineered devices using a novel aspiration assisted bioprinting process, which enables precise and high throughput bioprinting of human islets with controlled distance apart to tightly pack them in 3D. This maximizes the islet loading density within a given volume of device without aggregation of islets as aggregation induces significant hypoxia. In order to induce vascularization and keep bioprinted islets oxygenized until vascularization takes place, we bioprint human CD34+ vascular progenitor-derived endothelial cells and oxygen generating polycaprolactone microparticles, respectively. The current approach represents a major step toward scalable fabrication of an artificial pancreas for Type-I diabetes.